Investigative algorithms for disorders affecting plasma proteins with a focus on albumin and the calculated globulin fraction: a narrative review
Introduction
The measurement of total protein is one of the most requested tests within the biochemistry laboratory and is routinely carried out on an array of different biological fluids. Serum total protein refers to a quantitative determination of all proteins present excluding the clotting factors. Serum samples principally contain about 60–80 g/L of protein in healthy basal states and an approach to the diagnosis of high or low concentrations will be presented to aid test selection for diagnosis (1). We present this article in accordance with the Narrative Review reporting checklist (available at https://jlpm.amegroups.com/article/view/10.21037/jlpm-23-15/rc).
Methods
The narrative literature review was created by searching Medline, Google Scholar, OMIM and seminal texts over the period December 2022 to January 2023. The diagnostic algorithms were then created based on the literature review. The language was restricted to English. For further information please see Table S1.
Results
Plasma proteins
Plasma contains many proteins, which have a variety of roles and reflect the state of the tissues. While over 12,000 individual proteins have been characterised within the serum, the two major protein components are albumin and immunoglobulins (Figure 1) (2). Albumin is a protein exclusively synthesised by the liver whereas immunoglobulins are glycoproteins synthesised by B lymphocytes of the humoral immune system. Many other proteins are included in the determination of total serum protein, such as transferrin, α2-macroglobulin, and complement proteins. None of these individually contribute more than 5% of the total and so do not significantly affect total protein concentrations (3). Therefore serum total protein measurements are not diagnostic for a particular condition (4). Albumin is commonly measured in tandem with serum total protein. Generally, the laboratory will also calculate the globulin fraction:
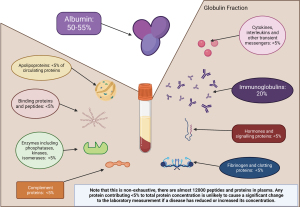
Eq. [1] used to calculate the globulin fraction using the total protein and albumin concentration (all values should be in the same units e.g., g/L) (3).
Albumin
Albumin is synthesised in the liver (Figure 2) accounting for 55–60% of the serum protein mass (5). Dietary protein intake varies widely but should not exceed about 2 g/kg of body weight/day (6). Albumin is a water-soluble 585 amino acid peptide chain, with a turnover time of 25 days and a weight of 65 kDa (7). It distributes between the intravascular (30–40%) and extravascular spaces (60%) predominately with significant pools in the lymph and skin (8). Healthy individuals lose 6% of their albumin via the kidneys and 10% via the gut, per day (7). Although albumin is freely filtered in the kidneys (9), its anionic charge limits this and it is actively reabsorbed in the proximal tubules. In health, a urinary albumin creatinine ratio is less than 3 mg/mmol (8). Albumin has many functions including the regulation of osmotic pressure, buffering of plasma pH, transport of hormones and more (Table 1) (10-17).
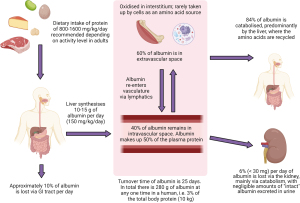
Table 1
Role | Details of function |
---|---|
Regulation of osmotic pressure | Major component (80%) of plasma oncotic pressure |
Transport of metabolites and ions | Transports molecules (e.g., free fatty acids, unconjugated bile acids, bilirubin) to the liver for metabolism and storage. Binding may regulate activity, clearance, or act as a reservoir e.g., calcium ions |
Binding and transport of hormones | Binds hormones including those with specific transport proteins e.g., thyroxine, cortisol, vitamin D and testosterone |
Binding and transport of drugs | Some drugs are protein bound therefore albumin concentration affects their pharmaco-kinetics and -dynamics e.g., phenytoin |
Buffering plasma pH | Reservoir donating and binding H+ ions |
Scavenging reactive oxygen species | Albumin is thought to be responsible for approximately 50% of the antioxidant properties of plasma |
Reservoir of nitric oxide | Blood pressure regulation |
Anticoagulant effect | Prevents coagulation and platelet aggregation |
Reduces inflammation | Inhibits the actions of cytokines e.g., tumour necrosis factor alpha (TNF-α) and complement proteins |
Albumin synthesis is primarily thought to be regulated by alterations in the colloidal oncotic pressure rather than the albumin concentration (7). For example, an acute loss of albumin (7%) during plasmapheresis is slowly replaced by the liver increasing albumin synthesis by 25% over 30 days (18). Both insulin and growth hormone stimulate albumin synthesis (19-21). A protein meal stimulates synthesis (21) but is not related to the concentration of amino acids in the circulation (22). Mild acidosis reduces albumin synthesis (23) and catecholamines have been documented to increase synthesis (24).
Immunoglobulins
There are five classes of immunoglobulins, although only three are routinely measured i.e., immunoglobulin G (IgG), immunoglobulin A (IgA) and immunoglobulin M (IgM). The five classes are as follows, in descending concentration order: IgG (7.0–16.0 g/L), IgA (0.7–4.0 g/L), IgM (0.4–2.3 g/L), immunoglobulin D (IgD) (<152.7 mg/L), immunoglobulin E (IgE) (5–120 KU/L) (25-27). The ranges provided in brackets are a guide and will vary with certain factors i.e., age, sex, and methodology. Immunoglobulins can either be membrane-bound antigen receptors or a secreted product of the adaptive immune system.
The basic monomer structure consists of a pair of identical heavy and light-chains arranged into a Y shape via disulphide bonds (4). The heavy chain defines the isotype, light-chains are either lambda or kappa providing additional subgrouping as each isotype exists in a lambda and kappa form, i.e., IgG kappa and IgG lambda (28). In addition to this there are four subclasses for IgG and two subclasses of IgA (29). An in-depth review of immunoglobulin synthesis and metabolism is outside the scope of this algorithm, but is summarised in Figure 3 (30-34).
Laboratory techniques
In the following section some of the commonly used analytical methods will be discussed.
Total protein method
Total protein methods include chemical methods (such as the biuret reaction), turbidimetry, direct optical methods, dye-binding methods, refractometry, capillary electrophoresis, western blotting, mass-spectrometry, immunofixation electrophoresis, Kjeldahl method, Lowry method, and nephelometry (3). The biuret reaction is one of the most commonly available techniques with good linearity between 15 to 120 g/L (3,35). Interferences include ethylenediaminetetraacetic acid, sucrose, phospholipids, glycerol, triton X-100, and dithiothreitol (36). Hyperbilirubinaemia induces a negative interference causing pseudohypoprotinaemia and pseudohypoglobulinaemia (37). Spuriously raised protein concentrations can be produced by turbid specimens or those with particulates within the serum as well as moderate to marked haemolysis and lipaemia (38).
Albumin method
The most commonly available albumin assays are dye based (bromocresol green or purple) spectrophotometry methods (39). Other methods include immunonephelometric, immunoturbidometric techniques, and conventional, capillary serum protein electrophoresis (40,41). Reasons for possible spurious results related to method selection are summarized in Table 2. Spurious results can also be caused by taking blood samples from an arm attached to an intravenous drip (dilution artefact), or contamination with blood tube additives e.g., sodium citrate contamination (dilutional drop in total protein, as well as other characteristic changes such as hypocalcemia) (42).
Table 2
Test name | Factors that can affect the reliability of the results |
---|---|
Bromocresol green dye | Can give a falsely high reading in hypoalbuminemia and a falsely low reading in hyperalbuminemia; poor performance if albumin <20 g/L; low specificity, other proteins interfere |
Bromocresol purple dye | Falsely low when the albumin, and albumin: globulin ratio, is low (nephrotic syndrome); more specific with less protein interference than bromocresol green, but more difficult to calibrate; heparin negatively interferes |
Immunoassay | Imprecise and weak reactions from albumin fragments causing falsely high readings; not commonly used |
Serum electrophoresis | Falsely high albumin if other proteins cluster around the densitometry peak (e.g., pre-albumin and α1 acid glycoprotein); protein can be absorbed by the matrix (e.g., the paper) and give falsely low readings; high interlaboratory variation |
Calculated globulin fraction {Eq. [1]}
The globulin fraction is a calculated metric produced from the subtraction of albumin from the total protein. This fraction consists of immunoglobulins (30%) with enzymes, carrier and complement proteins, among others, contributing to the remaining 70% (Figure 1) (4). Globulin fraction cut-offs are often locally decided by laboratories i.e., (20–39 g/L), poor consensus on this cut-off is thought to arise due to variation in methodology performance and local population differences (43). A clear lower limit for globulin is required to reduce the current 5- to 6-year delay there is on detecting primary hypogammaglobulinaemia (43-47). A few studies have suggested that a globulin less than 18 g/L (children ≥10 years and not under haematology or oncology review) should indicate screening for hypogammaglobulinaemia, but there is less certainty in regard to adult thresholds (43,44). The globulin fraction in hypogammaglobinaemia, may also be spuriously normal in the acute phase response, due to the rise in concentration of other proteins (43).
Measurement methods for serum immunoglobulin
Immunoglobulins are measured as part of total protein quantification, and during serum electrophoresis and immunofixation. Direct individual measurement of the separate subclasses can be carried out by automated nephelometry or sandwich enzyme-linked immunosorbent assays (ELISA). Specific immunoglobulins can also be measured, but are outside the scope of this paper (4,48,49). Immunoglobulin isotype assays can be subject to prozone/antigen excess errors. Monoclonal proteins, in particular, can result in non-linearity and misreporting. Nephelometry methods are affected by turbid samples or particulates (48,50). Mass spectrometry, a highly specific and sensitive method, is currently not yet widely used (51).
Serum electrophoresis
Serum electrophoresis segregates proteins into six fractions based on size, shape, and electrical charge (48). Traditionally this was carried out on agarose gel, which has been mostly replaced by capillary zone electrophoresis (CZE) (3). Interpretation of electrophoretograms is complex and requires specialist training (48). Traditional gel electrophoresis is subject to point of application artifacts with some precipitants unable to migrate into the gel, such as euglobulin and cryoprecipites, which may contain monoclonal proteins leading to spuriously negative results. Discrepancies in interpretation and reporting may also occur and rarely low concentrations of monoclonal proteins are missed (3).
Serum free light-chains
Free circulating kappa and lambda light-chain components within the serum can be used as a diagnostic marker for monoclonal proteins (52). Lambda concentrations in health are twice that of kappa due to kappa’s smaller molecular weight and thus increased renal filtration (48). Serum free light-chains analysis is more sensitive (sensitivity: 0.76, specificity: 0.96) than urine Bence Jones protein quantification (monoclonal globulin protein or immunoglobulin light chain in the urine), as the rate of paraprotein synthesis needs to exceed the renal metabolism rate before being detectable in urine (53,54). However, it is argued that urine light-chain assessment is more specific than serum free light-chain analysis (as it quantifies all/polyclonal free light-chains, as well as monoclonal, which may result in a spuriously normal kappa: lambda ratio) (55).
The Freelight® immunoturbidimetric assay is a latex-enhanced conjugated polyclonal antibody nephelometric assay (56). Other methods include immunonephelometric assays or sandwich ELISA kits (3). Serum free light-chain assays are very prone to prozone antigen excess errors and cross-reactions. The prozone error/antigen excess is a reaction kinetic error where there is such a high concentration of the target analyte this causes incomplete reagent antibody binding, a falsely low concentration (due to a diminished signal), and is overcome by diluting the sample (48,57). This assay type is also prone to non-linear results, discrepancies in dilution, and polymerisation effects. Some patients may also have a unique light-chain epitope that is not recognised (52,57).
Urine electrophoresis
Urine electrophoresis can characterise urinary proteins and detect Bence Jones protein, which can be typed by immunofixation (48). Urine electrophoresis is generally carried out via a 2D gel electrophoresis method, applying a high electric current to a high-resolution agarose gel from a commercial kit or via CZE (58,59) and is subject to the same analytical errors as serum (3). It is time-consuming and, if the urine is too dilute, it may require a concentration step or a repeat sample. The quantification of urine protein is normally carried out on densitometry, which can be inaccurate and is dependent on the measurement of urine total protein. This is often measured by a dye binding method, which has its own inaccuracies as discussed above. In urine electrophoresis monoclonal bands however can be defined from the polyclonal background (55).
Other tests
Most liver function and bone profiles consist of albumin and total protein. The other parts of these profiles will be discussed in the companion articles in these series.
Urine protein creatinine ratio (PCR) uses creatinine to correct for diurnal variation in urine concentration. Urine PCR is a fast, simple way of detecting if proteinuria is present, and if so, to what degree (60-62). The most commonly reported thresholds for a random PCR sample are >20 mg/mmol (>0.2 mg/mg) for detecting proteinuria and >350 mg/mmol (>3.5 mg/mg) for nephrotic range proteinuria (62).
Urine albumin creatinine ratios (ACR) are often recommended instead of PCR as this test has greater sensitivity for low levels of proteinuria but can miss cases of proteinuria without albuminuria. For example, urine PCR testing has greater utility over ACR when screening for pre-eclampsia in pregnancy (63). Urine albumin and creatinine are generally measured using immunonephelometry, immunoturbidimetry, and occasionally high-performance liquid chromatography. Urinary albumin can be carried out at the bedside via dipstick testing, although this method is considered to have a false negative rate (around 40%) and is not quantitative (64).
Albumin present in trace amounts, microalbumin, can point to nephropathy (65) but false positives can occur e.g., urinary tract infections, intercurrent illnesses and exercise (65,66). Initial positive results should be repeated within 2–12 months and a mid-stream urine sent for microbiology cultures (66). The most commonly quoted reference ranges for urine albumin: creatinine ratio is from Kidney Disease: Improving Global Outcomes (KDIGO) program and are as follows: <3 mg/mmol normal, 3–30 mg/mmol moderate increase, >30 mg/mmol severely increased urine protein excretion, and >220 mg/mmol nephrotic syndrome (67).
Detection of α1 antitrypsin in stool, or albumin scintigraphy, may be used to screen for excess gastrointestinal loss of protein (68-70). Enzyme linked immunoassay quantification of faecal α1 antitrypsin is the most used and reliable method of diagnosis of protein losing enteropathy (PLE) (71-75). Additional tests, such as serum anti transglutaminase IgA, faecal cultures/microscopy, faecal calprotectin, imaging studies, and intestinal biopsies, should be considered to elucidate the cause and hence treatment strategy of PLE (75).
Natriuretic peptides are produced by a failing heart (causing a dilutional state and low protein) (4,76). The measurement of a pre-cursor prohormone N-terminal pro-hormone brain natriuretic peptide (NT-proBNP) is more commonly measured than BNP as it has a longer half-life and better stability profile (77). The quoted NT-proBNP cut-offs for referral are 400–2,000 ng/L indicating echocardiography within 6 weeks for a new case, and >2,000 ng/L requiring urgent referral (78). Others suggest a lower threshold of >125 ng/L (79). Note pg/mL is equivalent to ng/L.
BNP or NT-proBNP are often measured by immunoassays and may be subject to interference with biotin, human anti-animal or heterophilic antibodies, prozone/antigen excess, and rarely macro complexes (80). BNP and NT-proBNP are non-specific e.g., raised in sepsis, older age (>70 years), renal failure (eGFR <60), exercise, cirrhosis, ventricular overload, acute coronary syndrome, hypoxaemia, etc. Low values can be seen with obesity, diuretics, angiotensin-converting enzyme inhibitors, angiotensin receptor blockers and aldosterone antagonists (81,82).
Albumin can be measured on ascitic fluid samples to calculate the serum-ascitic albumin gradient. This is used in the differential diagnosis of ascites formation and a gradient of >11 g/L is suggestive of portal hypertension (83).
The serum-ascites albumin gradient formula is as follows (83):
Blood and ascitic samples should be collected simultaneously and measured in the same units. The original paper that defined this cut-off was not explicit in analytical method simply stating a commercial Abbott kit was used (84). Therefore, it would be prudent to verify and modify this cut-off depending on the local methods used.
Fluids (other than serum and urine) tend not to have a consistent albumin composition and results may have high variability. The main methods used for fluid albumin measurement include dye binding methods (bromocresol purple and green) or immunoturbidimetry. The bromocresol green method has been found to have a positive bias of 1–3 g/L when compared to immunoturbidimetry. Dye binding methods in fluid can have a positive bias compared with serum which may lead to a significantly lower serum-ascitic albumin gradient threshold (85).
Hypoproteinaemia
Normal serum total protein measurement is reported to range from 60 to 80 g/L (1,86). Therefore 59 g/L and below defines hypoproteinaemia (87,88). Hypoproteinaemia can present with a spectrum of symptoms including muscle wasting, hair loss, oedematous limbs, failure to thrive, infections, fatigue (4), and causes are multiple (Table 3) (88). Hypoproteinaemia occurs principally due to decreases in albumin and immunoglobulin (particularly if affecting IgG) (4). Most causes can be identified by history and examination e.g., pregnancy and sepsis, however diagnostic algorithms are presented below for hypoalbuminaemia and hypogammaglobinaemia.
Table 3
General cause | Subtype | Example |
---|---|---|
Dilutional states | Fluid overload/oedema | Cardiac failure |
Cirrhosis | ||
Nephrotic syndrome | ||
Third trimester pregnancy | ||
Iatrogenic | Intravenous fluid | |
Physiological stress | Starvation | Kwashiorkor |
Marasmus | ||
Genetic disease | Cystic fibrosis | |
Chronic intestinal disease | Coeliac disease | |
Pernicious anaemia | ||
Age | Frailty (elderly) | |
Protein loss | Fluid imbalance and loss | Haemorrhage |
Peritoneal effusion | ||
Pleural effusion | ||
Intestinal disease | Protein losing enteropathy | |
Ulcerative colitis | ||
Exudative skin disease | Severe burns, erythroderma | |
Renal disease | Chronic kidney disease | |
Diabetes nephropathy | ||
Nephrotic syndrome | ||
Renal cell carcinoma | ||
Decreased synthesis | Humoral immunodeficiency | Bone marrow failure |
Common variable immunodeficiency | ||
Hypogammaglobulinaemia | ||
Liver disease | Alcoholic liver disease | |
Chronic hepatitis | ||
Cirrhosis | ||
Cachexia | Malignancies | |
Inflammation | Bacterial infection | |
Sepsis | ||
Severe trauma |
Hypoalbuminaemia
Hypoalbuminaemia causes fluid shifts therefore peripheral or pulmonary oedema, and ascites; an algorithm for a laboratory approach to its investigation is shown in Figure 4. Low serum albumin concentration is associated with poorer prognosis during illness (97-99). However, albumin supplements do not significantly affect outcomes (100,101). Albumin decreases in states of inflammation and is thus a negative acute phase protein (91), partly as increased capillary permeability leads to albumin extravasation that outstrips the rate of albumin synthesis (3).
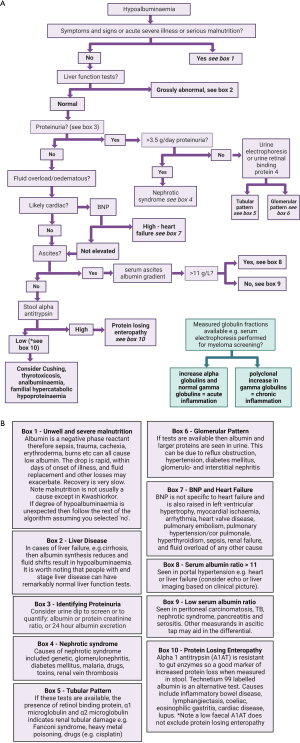
Only severe malnutrition states can lead to protein deficiencies, such as kwashiorkor and marasmus (102,103). Kwashiorkor primarily affects children and arises from a predominant protein deficiency and marasmus is a global deficiency of macronutrients including protein, lipids, and carbohydrates (102-105).
Albumin may be used, with extreme caution, as one of the indicators of nutritional status in patients with severe gastrointestinal disease on supported nutrition, as well as those at risk of refeeding and cancer patients, but many nutritional assessment and screening tools do not include protein nor albumin concentrations (91,106). In patients with simple starvation the rate of albumin catabolism and synthesis falls so albumin remains normal despite a prolonged state of malnutrition e.g., concentrations are normal in anorexia nervosa (106,107).
Albumin may reduce with age, likely a reflection of the extent of physiological stress from underlying age-related inflammatory conditions rather than a long-term indicator of nutritional status (108,109). During sepsis and general physiological stress, albumin extravasation increases, and the liver shifts synthetic function to inflammatory, and other, proteins thereby reducing albumin synthesis. Depending on the severity, the source, and progression of healing, it may take 3–4 months for albumin concentrations to normalise despite only taking 3–7 days to decrease post the initial inflammatory insult (109). C-reactive protein (CRP), erythrocyte sedimentation rate (ESR) and procalcitonin may help elucidate the cause of the active phase response depending on local availability and condition specific pathways (110).
Fluid imbalances in the extracellular plasma compartment can cause dilution of the plasma proteins leading to low serum total protein e.g., cardiac failure, liver failure and nephrotic syndrome. However, these conditions are also associated with cachexia, malnutrition, inflammation, liver dysfunction, PLE, increased extravascular loss and haemodilution (10). Spurious results can be due to intravenous drip fluid contamination, as discussed earlier, (111) and so if the results unexpected consider repeating the venesection (3,111).
Liver disease, when severe and chronic, causes hypoalbuminaemia. Cirrhosis, for example, can lead to a reduced albumin production by 60–80%, a feature further exacerbated by dilution, shift of albumin to a different compartment, and post transcriptional changes to albumin (112). Acute liver disease does not present with hypoalbuminaemia typically; for an algorithm on the interpretation of abnormal liver function tests please see other useful papers and the companion articles in this series (113).
Physiological stress suppresses albumin synthesis (114). Negative feedback from a high osmotic pressure will also decrease albumin synthesis, as can diabetes mellitus (insulin stimulates albumin gene expression) (115).
The autosomal recessively inherited condition analbuminaemia causes a complete lack of albumin (OMIM) (116). Heterozygotes are disease free and distinct bands are seen on serum electrophoresis (bisalbuminaemia or alloalbuminaemia) (48,116). Analbuminaemia is often asymptomatic but there can be perinatal problems due to shifts of fluid across the placenta (117). Depending on where the mutation is it may affect albumin’s ability to carry thyroid hormone, divalent copper or nickel ions, fatty acids and drugs, therefore may cause abnormal blood tests, if not morbidity, due to reduced function, especially in the lipid pathway (16,116,118). Analbuminaemia may also lead to a degree of pulmonary oedema and increased risk of respiratory infections leading to infant mortality (119). Symptoms of analbuminaemia are minimised by a compensatory increase in other circulating proteins (118).
Protein loss can occur through the gastrointestinal tract i.e., in PLE (any state in which oedema and increased lymphatic pressure adversely affects gastrointestinal function) e.g., inflammatory bowel disease, lymphangiectasis, coeliac, eosinophilic gastritis, cardiac disease and lupus (120-122). In PLE 60% of total albumin is lost via the gut along with indiscriminate loss of immunoglobulin, fibrinogen, lipoprotein, transferrin, and other proteins (68,69,75,123,124).
Proteinuria generally arises due to abnormal transglomerular passage of proteins due to the increased permeability of the glomerulus capillary walls or impaired absorptive capacity of the proximal tubules (125). Proteinuria is used as a biomarker of renal risk in the general population, non-diabetic chronic kidney disease (CKD) and diabetic CKD patients and in CKD staging criteria (63).
Significant loss of albumin from the kidneys occurs in nephrotic syndrome the causes are listed in Table 4 (8,126). End stage renal failure can result in the loss of 30–300 mg of albumin a day, but interpretation of laboratory results can be affected by fluid status and shifts (127). Dietary protein supplementation only increases the renal protein loss and does not increase the plasma albumin concentration (128). Usually the renal loss of albumin in diabetic nephropathy is not sufficient to cause hypoalbuminaemia unless the loss becomes nephrotic (7). However, microalbuminuria, seen in diabetes and hypertension, probably has a deleterious effects on the kidneys and accelerates the decline in renal function (8).
Table 4
Commonest causes in children | Commonest causes in adults |
---|---|
Idiopathic, primary, and genetic | Usually secondary: toxins, drugs, heavy metals, autoantibodies, post-infections antibody complexes (e.g., group B streptococcus) or, myeloma and neoplasm related immune complexes |
Biopsies normally show minimal change disease, membranoproliferative glomerulonephritis or focal segmental glomerulosclerosis |
During illness it is proposed tissue utilisation and catabolism of albumin increases thus providing amino acids for tissue repair (129). Biochemically altered albumin is taken into cells and catabolised, predominantly in muscle and skin, whereas unaltered albumin is released back into the extracellular fluid (9,130).
Various medication have been linked to hypoalbuminaemia including (131):
- Dapsone (only reported in long term use in dermatology (autoimmune bullous disorders); may reflect the extent of the skin or gut involvement but all cases recovered rapidly on cessation of dapsone) (132);
- Dexmedetomidine (used as a sedative in ITU so could be spurious);
- Micafungin (no case reports found, used to treat invasive fungal infections so may be spurious, i.e., caused by the infection);
- Glycerol phenylbutyrate (no case reports found, used to treat people with urea cycle defects on very low protein diets);
- Isavuconazole (no case reports found, used to treat invasive fungal infections so may be related to tissue inflammation).
Also, caution is required when prescribing certain drugs that bind protein in the case of hypoalbuminaemia e.g., benzodiazepines (131). Anti-tumour medication (e.g., cytotoxic, antimetabolites, tyrosine kinase inhibitors, immunotherapy, histone deacetylase inhibitors, proteasome inhibitors, topoisomerase inhibitors, VEGF and EGFR antibodies) is often associated with hypoalbuminaemia, but it is likely that the cancer, and tumour lysis, leads to fluid shifts and is the cause rather than the drugs themselves (133).
Hypogammaglobulinaemia
Humoral immunodeficiency refers to a spectrum of conditions that result in a deficiency of one or some of the classes of immunoglobulins (134). These conditions may be acquired or congenital and arise due to a malfunction of maturation of B-cell lymphocytes (47). Other reported causes of low calculated globulin fraction include, cirrhosis, kidney conditions such as nephrotic syndrome, malnutrition, acromegaly, and malignancies (135-137). For a diagnostic algorithm please see Figure 5.
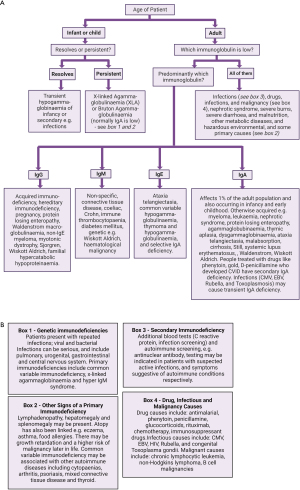
Primary immunodeficiencies arise from chromosomal or genetic abnormalities e.g., X-linked agammaglobulinemia, common variable immunodeficiency (CVID), transient hypogammaglobulinaemia, selective IgA deficiency and hyper IgM syndrome (43-45,47,48). The diagnostic work up for primary immunodeficiencies includes the measurement of serum immunoglobulins (IgG, IgM, and IgA), full blood count with white blood cell differential, flow cytometry for B-cell and T-cell evaluation as well as genetic testing, e.g., BTK gene mutations (43,44,48).
Common variable immunodeficiency (CVID) presents with hypogammaglobulinaemia and an increased susceptibility to bacterial infections and is one of the most common antibody deficiencies accounting for around 40% of primary immunodeficiencies in the western world (45,138). In CVID serum IgG is markedly reduced and, to a lesser and variable extent, so are IgA and/or IgM (138). Around 12% of those with CVID go on to develop chronic liver disease, therefore in liver disease cases with hypogammaglobinaemia some may be undiagnosed CVID (139). CVID mimics the symptoms of gastrointestinal diseases, and studies have confirmed there is a high prevalence of inflammatory, infectious and malignant gastrointestinal disorders (140). CVID patients may also develop haemolytic anaemia and malignancies due to reduced immune function (141,142).
Acquired causes of reduced immunoglobulin synthesis include malignancies, drugs, (discussed below), malnutrition, and environmental conditions such as ionising radiation exposure. Sometimes metabolic disorders such as propionic acidaemia can produce hypogammaglobinaemia, but it is unlikely that hypoproteinaemia would be the presenting feature (44,47,143-145). Patients with liver disease can present with both hypoglobulinaemia and hyperglobulinaemia depending on the aetiology of the organ damage, however the latter is much more common (4,112,146).
Severe malnutrition has diverse effects on the humoral immune system but decreased immunoglobulin synthesis can occur (95). Hypogammaglobinaemia can be observed with gastrointestinal conditions such as coeliac, pernicious anaemia, and irritable bowel disease (i.e., Crohn, ulcerative colitis) (147,148).
Infectious agents such as viruses, bacteria, or recovery from severe sepsis can cause immunoparesis (suppression of polyclonal immunoglobulins). Viral causes of hypogammaglobulinaemia include Epstein-Barr virus, cytomegalovirus, rubella, and human immunodeficiency virus (143). This phenomenon is also well documented in multiple myeloma patients; however, these patients do not tend to present with hypoproteinaemia due to the increased production of a monoclonal protein. Other haematological malignancies can also cause hypogammaglobinaemia, as can their treatment with stem cell transplantation (143,149).
Immunoglobulins will also be lost in nephrotic syndrome, in PLE, and in severe burns (143). There are a few reports in the literature of acromegaly or excessive growth hormone being associated with apparent hypoglobulinaemia or hypoproteinaemia (135,150,151). The pathophysiology behind this is unknown as growth hormone stimulates protein synthesis so may be altered protein metabolism or increased loss (151). Reports of hypoproteinaemia associated with acromegaly are sparse however and therefore it is unlikely that a low total protein would be a presenting symptom.
The British National Formulary (BNF) lists hypogammaglobulinaemia as a side effect of the following drugs: mycophenolate, tacrolimus, cyclosporin A, tafasitamab, diazoxide, daratumab, blinatumomab, carbamazepine, belatacept, rituximab and pencilliamine (131). Other drugs that have been associated with hypogammaglobulinaemia include corticosteroids, chemotherapy, valproic acid, phenytoin, sulfasalazine, chloroquine, fenclofenac, hydantoin, and lamotrigine (152,153).
Hyperproteinaemia
A total protein concentration of 81 g/L and above is considered to be hyperproteinaemic (1). Causes include dehydration, inflammation, autoimmune disease and bone marrow dysfunction (Table 5). Clinically it is difficult to distinguish the effects and symptoms of the primary disease from hyperproteinaemia (105). Symptoms and signs associated with hyperproteinaemia may include anaemia, renal dysfunction, liver dysfunction, or the laboratory phenomena of apparent hypercalcaemia, pseudohyponatremia, etc. (54,155). Renal involvement with monoclonal gammopathy of unknown significance (MGUS), for example, is a clinically important entity (156). MGUS of clinical significance, is a term used for when a disease pathology is caused by end organ build-up of the protein (157). These diseases can present to the dermatologist (e.g., finger vasculitic lesions caused by a cryogloblinaemia, ecchymoses of AL amyloidosis, urticaria caused by Schnitzler syndrome, xanthelasma like periorbital deposits of necrobiotic xanthogranulomas, tissue thickening of scleromyxoedema or the telangiectasis of the TEMPI syndrome); the neurologist (peripheral neuropathy caused by distal acquired demyelination or cerebellar ataxia of CANOMAD); the respiratory physician (in Clarkson disease or crystal storing histiocytosis); or the renal physician (glomerulonephritis or tubulopathy) to name a few (158). Rarely oncology patients, particularly those with Waldenstrom macroglobulinaemia, may develop hyperviscosity syndrome. This emergency can present with neurological deficits, mucosal bleeding, and visual disturbances (159).
Table 5
General cause | Subtype | Example |
---|---|---|
Plasma cell neoplasms and B-cell lymphoma | Plasma cell dyscrasia | Amyloidosis |
Monoclonal gammopathy of known significance | ||
Monoclonal gammopathy of clinical significance | ||
Monoclonal gammopathy of renal significance | ||
Multiple myeloma | ||
Plasma cell leukaemia | ||
Waldenstrom macroglobulinemia | ||
Fluid imbalance | Dehydration | Addisonian crisis |
Cholera | ||
Diabetic ketoacidosis | ||
Severe vomiting and diarrhoea | ||
Very poor intake | ||
Inflammatory | Autoimmune disease | Autoimmune hepatitis |
Systemic lupus erythematosus | ||
Rheumatoid arthritis | ||
Sjogren | ||
Infective | Hepatitis | |
HIV infection | ||
Tuberculosis | ||
Sepsis |
HIV, human immunodeficiency virus.
Hyperalbuminaemia
If the tourniquet or cuff is on a limb for a long period during venesection, then one can get a falsely raised albumin concentration in the serum (3). If there is any doubt repeat the sampling. The most common reason, and many argue the only reason, for hyperalbuminaemia is dehydration. Drugs that shift body fluid balance may alter albumin concentration. Cisplatin has also been linked to hyperalbuminaemia due to nephrotoxicity (160). Hyperalbuminaemia has been reported with the use of co-magaldrox (aluminium hydroxide and magnesium hydroxide) and simeticone with aluminium hydroxide and magnesium hydroxide (131), however there are no case reports in the literature and perhaps the metal salts are interfering with the laboratory techniques used to measure albumin in the laboratory (161).
Retinol drugs, commonly used in dermatology for acne, psoriasis, hyperkeratotic disorders and cutaneous lymphomas, up-regulate albumin production and have been linked to hyperalbuminaemia (162). Diet may have a small effect and extreme, protein-heavy diets may lead to hyperalbuminaemia (163).
Hypergammaglobulinaemia
An algorithm for a laboratory approach to its investigation is shown in Figure 6. A cut-off of 45 g/L is commonly used (164-166). This threshold has a sensitivity of 73.15%, specificity 32.14%, positive predictive value of 44.12% and negative predictive value of 62.1% for detecting the presence of paraproteins in hospital patients (164). Ranges should be method and population dependent. The most important point for clarification, with hypergammaglobinumaemia, is whether the rise is polyclonal or monoclonal.
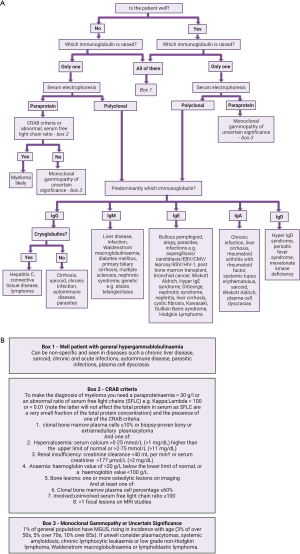
Polyclonal hypergammaglobulinaemia
Inflammation causes a polyclonal hypergammaglobulinaemia. Inflammatory symptoms can be broad and nonspecific and include headaches, chills, fever, loss of appetite, and muscle stiffness etc. (4). A raised globulin fraction can be seen in many autoimmune and vascular diseases (167,168). Raised polyclonal IgG responses are associated with vasculitis, autoimmune diseases, infections, IgG4-related diseases, and iatrogenic sources such as intravenous immunoglobulins. Elevations in IgA are associated with vasculitis, HIV infections, autoimmune diseases such as SLE, rheumatoid arthritis, coeliac disease, and IgA nephropathy. Polyclonal elevation of IgM can be associated with immunoglobulin class-switching disorders, acute infection, and hyper-IgM syndrome. IgD and IgE polyclonal elevations are rare, but IgD has been associated with hyper-IgD syndrome, and IgE is associated with asthma, allergies, atopy, lymphoma, parasite infections and hyper-IgE syndromes (144,169).
Further testing depends on the suspected condition, autoimmune serology testing should not be requested indiscriminately but based on the clinical presentation and history. For example, suspected inflammatory bowel disease may indicate tests including CRP, full blood count, anti-tissue transglutaminase antibodies, thyroid function, stool cultures and faecal calprotectin or immunochemical testing (168,170,171). Suspected SLE may indicate more general tests such as CRP, ESR, full blood count and a staged approach to specific immunology tests including anti-nuclear antibodies, complement (C3 and C4), anti-phospholipid antibodies and the follow-on tests of anti-double stranded DNA or extractible nuclear antigens (172).
Infective agents such as bacteria and viruses will produce an immune response resulting in a polyclonal antibody response, which may result in hyperproteinaemia and hypogammaglobinaemia. Specific samples may include HIV, infective exacerbation COPD, infective exacerbation of bronchiectasis, syphilis, bacterial endocarditis, mycetoma, acute rheumatic fever, cholecystitis, malaria, etc. (164).
Immunoglobulins are generally raised as part of the picture of liver damage. A raised IgM has been associated with primary biliary cirrhosis, raised IgA with alcoholic injury and portal cirrhosis and IgG was generally raised in chronic active hepatitis. As discussed, liver patients can also have a low albumin due to reduced synthetic function of the liver.
Non-haematological malignancies that may have polyclonal hypergammaglobulinaemia include liver, kidney, lung and ovarian (173). Non-haematological malignancies tend to have a raised IgG concentration; however, the neoplasm needs to induce inflammation to see a polyclonal response (169).
IgG subtypes can be individually raised e.g., IgG4 in a fibroinflammatory disorder, which may present with a raised total protein, calculated globulin fraction, polyclonal IgG and signs such as lymphadenopathy, and laboratory findings such as eosinophilia, etc. (169). It is difficult to diagnose due to non-specific symptoms, ethnic and sex variation in IgG4 and it remains a relatively rarely diagnosed disease (174,175).
Monoclonal gammopathy
A broad spectrum of haematological malignancies may have a monoclonal gammopathy, which may present with a raised globulin fraction. The CRAB criteria are used to help distinguish diagnoses (53). Haematological malignancies may include multiple myeloma, Waldenström macroglobulinaemia, acute myeloid leukaemia, chronic lymphoid leukaemia, chronic myeloid leukaemia (164). MGUS is benign but can progress and develop into multiple myeloma. MGUS of renal or clinical significance refers to diseases where the protein deposition in organs causes a variety of clinical presentations (see above). A paraprotein should stimulate further investigation (Table 6).
Table 6
Disease | Monoclonal protein band | Distinctive features |
---|---|---|
MGUS | Paraprotein band <30 g/L | No evidence of end organ damage (aka anaemia, renal failure, hypercalcaemia) |
No evidence lytic lesions on X-ray | ||
<10% clonal plasma cell in bone marrow | ||
Multiple myeloma | Paraprotein band ≥30 g/L | Presence of paraprotein in the urine |
Evidence of end organ damage: anaemia, hypercalcaemia, renal disease, osteoporosis | ||
Evidence of lytic lesions on X-ray | ||
≥10% clonal plasma cell in bone marrow | ||
IgA ≥15 g/L | Note that IgA myeloma may present with lower paraprotein band, cut will still need to meet the CRAB criteria | |
No or trace paraprotein, abnormal light chain ratio | Light chain myeloma (will still need to meet the CRAB criteria); rule out cyroglobinaemia (false negative) if clinical suspicion high (keep sample warm); a secretory myeloma, diagnosis still relies on the CRAB criteria for the other features | |
Smouldering myeloma | Paraprotein band ≥30 g/L | No evidence of end organ damage (aka anaemia, renal failure, hypercalcaemia) |
Urine monoclonal protein ≥500 mg/24 h | No evidence of lytic lesions on X-ray | |
10–60% clonal bone marrow plasma cells | ||
Waldenstrom macroglobulinemia | IgM paraprotein | Evidence of hyper-viscosity, enlarged spleen and/or liver, anaemia, and swollen lymph nodes |
MGUS, monoclonal gammopathy of unknown significance; CRAB, Calcium elevation, Renal dysfunction, Anaemia and Bone disease.
Bence Jones proteins can be present in the urine of MGUS patients, but it is less frequent, and they generally present in very small quantities (3,4,53,56,164,173).
High concentration of protein, particularly those of an IgM paraprotein source, or of rheumatoid factor, are a common listed interferent on many different commercial assay’s kit inserts. It is also well documented that particularly hyperparaproteinaemia can cause a pseudohypophosphataemia and pseudohyponatremia sometimes seen in multiple myeloma patients (3,4,176).
Generally, there are more drugs that are associated with hypogammaglobulinaemia than hyperglobulinaemia. Drugs associated with hyperglobulinaemia include amiodarone, intravenous immunoglobulins, and drugs that can induce a drug-related autoimmune disease (177,178). Biological drugs such as daratumumab can be picked up as a monoclonal band on serum electrophoresis. As more biological antibody drugs are introduced this could become a larger problem. Good communication between the clinicians and the laboratory are required to avoid misclassifying the patient’s therapeutics as new monoclonal bands (179).
Special states, e.g., pregnancy and childhood
Albumin in pregnancy
Haemodilution can also occur as part of normal physiological processes such as pregnancy, where plasma volume on average expands by 45% (33). During pregnancy and lactation there is also a chronic but mild inflammatory response increasing the vascular permeability and reducing albumin concentration (180,181). Hypoalbuminaemia is a common finding in pregnancy during the third trimester and should not be over investigated (182).
During breast feeding there does not seem to be a serum albumin difference between mothers who may be assumed to be malnourished compared to those considered to be well nourished (183). Studies have not demonstrated any effect of lactation in a large systematic review (184).
Albumin in childhood
In prematurity albumin is associated with gestational age and children born under 32 weeks have an average of albumin of 30.6±4.7 g/L (184). From early infancy over childhood albumin concentrations rise (185,186). Therefore, age-related reference intervals should be employed such as those from the Canadian Laboratory Initiative on Paediatric Reference Intervals (CALIPER) database (186).
Immunoglobulin in pregnancy
During human pregnancy there is a shift from TH1 immune response into a TH2 immune response and dynamic changes in the B-cell development of immunoglobulin profile are thought to occur. There is a significant decrease in the concentration of transitional B-cells within whole blood during pregnancy (187). IgG starts to mildly decrease during the second trimester due to haemodilution. IgM, IgA and IgE concentrations vary between individual pregnancies and have been observed to stay consistent as well as increase and decrease (27,188,189).
Immunoglobulins in childhood
Immunological changes within infancy throughout childhood to adolescence are diverse and complex. A full review is outside the scope of this paper, but the summarised expected changes within immunoglobulin concentrations are as follows: IgG concentration increases 18% per year until the age of five, where the rate of increase slows to 2% per year. IgA is on average higher in females than male infants, IgA increases by about 27% per year for the first 7 years of life before plateauing. IgM rapidly increases during the first year of life but tapers off past this point (190-192). Therefore, age-related reference intervals should be employed such as those from the Canadian Laboratory Initiative on Paediatric Reference Intervals (CALIPER) database (186).
Conclusions
Protein, albumin, and globulin is a useful screening test for detecting protein imbalances within the body, however the investigation can be challenging with numerous causes. Many factors need to be considered when interpreting these tests including acute phase/inflammatory response, hydration status and organ disease. A set of diagnostic algorithms have been created to guide the reader’s approach and provide a systematic route of testing. Each patient is unique however and the clinical picture may direct the reader to skip steps or refer other algorithms within the series or in the literature. These algorithms are not a replacement for experience, expert opinion or local guidelines and should instead act as a diagnostic aid.
Acknowledgments
Funding: None.
Footnote
Provenance and Peer Review: This article was commissioned by the editorial office, Journal of Laboratory and Precision Medicine for the series “Investigative Algorithms in Laboratory Medicine II: Focus on Bone and Liver”. The article has undergone external peer review.
Reporting Checklist: The authors have completed the Narrative Review reporting checklist. Available at https://jlpm.amegroups.com/article/view/10.21037/jlpm-23-15/rc
Peer Review File: Available at https://jlpm.amegroups.com/article/view/10.21037/jlpm-23-15/prf
Conflicts of Interest: All authors have completed the ICMJE uniform disclosure form (available at https://jlpm.amegroups.com/article/view/10.21037/jlpm-23-15/coif). The series “Investigative Algorithms in Laboratory Medicine II: Focus on Bone and Liver” was commissioned by the editorial office without any funding or sponsorship. ARS received an honoraria for being editor in chief of Clinical and Experimental Dermatology. KES declares the role as Deputy managing editor of Lab Tests Online (UK) and received the honoraria from Amgen and Daiichi Sankyo. KES served as the unpaid Guest Editor of the series. The authors have no other conflicts of interest to declare.
Ethical Statement: The authors are accountable for all aspects of the work in ensuring that questions related to the accuracy or integrity of any part of the work are appropriately investigated and resolved.
Open Access Statement: This is an Open Access article distributed in accordance with the Creative Commons Attribution-NonCommercial-NoDerivs 4.0 International License (CC BY-NC-ND 4.0), which permits the non-commercial replication and distribution of the article with the strict proviso that no changes or edits are made and the original work is properly cited (including links to both the formal publication through the relevant DOI and the license). See: https://creativecommons.org/licenses/by-nc-nd/4.0/.
References
- Berg J, Lane V. Pathology Harmony; a pragmatic and scientific approach to unfounded variation in the clinical laboratory. Ann Clin Biochem 2011;48:195-7. [Crossref] [PubMed]
- Saha S, Harrison SH, Shen C, et al. HIP2: an online database of human plasma proteins from healthy individuals. BMC Med Genomics 2008;1:12. [Crossref] [PubMed]
- Nadar R, editor. Tietz Textbook of Laboratory Medicine. 7th ed. St Louis, Missouri: Elsevier Health Sciences, 2022.
- Marshall WJA, Lapsley M, Day AP, et al. Clinical biochemistry: metabolic and clinical aspects. Amsterdam: Elsevier; 2014.
- Weaving G, Batstone GF, Jones RG. Age and sex variation in serum albumin concentration: an observational study. Ann Clin Biochem 2016;53:106-11. [Crossref] [PubMed]
- Wu G. Dietary protein intake and human health. Food Funct 2016;7:1251-65. [Crossref] [PubMed]
- Levitt DG, Levitt MD. Human serum albumin homeostasis: a new look at the roles of synthesis, catabolism, renal and gastrointestinal excretion, and the clinical value of serum albumin measurements. Int J Gen Med 2016;9:229-55. [Crossref] [PubMed]
- Bruschi M, Santucci L, Candiano G, et al. Albumin heterogeneity in low-abundance fluids. The case of urine and cerebro-spinal fluid. Biochim Biophys Acta 2013;1830:5503-8. [Crossref] [PubMed]
- Molitoris BA, Sandoval RM, Yadav SPS, et al. Albumin uptake and processing by the proximal tubule: physiological, pathological, and therapeutic implications. Physiol Rev 2022;102:1625-67. [Crossref] [PubMed]
- Gounden V, Vashisht R, Jialal I. Hypoalbuminemia. 2023.
- Ha CE, Bhagavan NV. Novel insights into the pleiotropic effects of human serum albumin in health and disease. Biochim Biophys Acta 2013;1830:5486-93. [Crossref] [PubMed]
- Bal W, Sokołowska M, Kurowska E, et al. Binding of transition metal ions to albumin: sites, affinities and rates. Biochim Biophys Acta 2013;1830:5444-55. [Crossref] [PubMed]
- Kendall FE. Studies on human serum proteins: II. crystallization of human serum albumin. J Biol Chem 1941;138:97-109. [Crossref]
- Wang ZM, Ho JX, Ruble JR, et al. Structural studies of several clinically important oncology drugs in complex with human serum albumin. Biochim Biophys Acta 2013;1830:5356-74. [Crossref] [PubMed]
- Hamilton JA. NMR reveals molecular interactions and dynamics of fatty acid binding to albumin. Biochim Biophys Acta 2013;1830:5418-26. [Crossref] [PubMed]
- Kragh-Hansen U. Structure and ligand binding properties of human serum albumin. Dan Med Bull 1990;37:57-84. [PubMed]
- Anraku M, Chuang VT, Maruyama T, et al. Redox properties of serum albumin. Biochim Biophys Acta 2013;1830:5465-72. [Crossref] [PubMed]
- Andersen SB, Rossing N. Metabolism of Albumin and γG-Globulin during Albumin Infusions and during Plasmapheresis. Scand J Clin Lab Invest Suppl 1967;20:183-4. [Crossref]
- Peavy DE, Taylor JM, Jefferson LS. Time course of changes in albumin synthesis and mRNA in diabetic and insulin-treated diabetic rats. Am J Physiol 1985;248:E656-63. [PubMed]
- Moshage HJ, de Haard HJ, Princen HM, et al. The influence of glucocorticoid on albumin synthesis and its messenger RNA in rat in vivo and in hepatocyte suspension culture. Biochim Biophys Acta 1985;824:27-33. [Crossref] [PubMed]
- Kernoff LM, Pimstone BL, Solomon J, et al. The effect of hypophysectomy and growth hormone replacement on albumin synthesis and catabolism in the rat. Biochem J 1971;124:529-35. [Crossref] [PubMed]
- Sun X, Kaysen GA. Albumin and transferrin synthesis are increased in H4 cells by serum from analbuminemic or nephrotic rats. Kidney Int 1994;45:1381-7. [Crossref] [PubMed]
- De Feo P, Horber FF, Haymond MW. Meal stimulation of albumin synthesis: a significant contributor to whole body protein synthesis in humans. Am J Physiol 1992;263:E794-9. [PubMed]
- Ballmer PE, McNurlan MA, Hulter HN, et al. Chronic metabolic acidosis decreases albumin synthesis and induces negative nitrogen balance in humans. J Clin Invest 1995;95:39-45. [Crossref] [PubMed]
- Bengtén E, Wilson M, Miller N, et al. Immunoglobulin isotypes: structure, function, and genetics. Curr Top Microbiol Immunol 2000;248:189-219. [Crossref] [PubMed]
- Khan SR, Chaker L, Ikram MA, et al. Determinants and Reference Ranges of Serum Immunoglobulins in Middle-Aged and Elderly Individuals: a Population-Based Study. J Clin Immunol 2021;41:1902-14. [Crossref] [PubMed]
- Amino N, Tanizawa O, Miyai K, et al. Changes of serum immunoglobulins IgG, IgA, IgM, and IgE during pregnancy. Obstet Gynecol 1978;52:415-20. [PubMed]
- Yu J, Song Y, Tian W. How to select IgG subclasses in developing anti-tumor therapeutic antibodies. J Hematol Oncol 2020;13:45. [Crossref] [PubMed]
- Nezlin R. Dynamic Aspects of the Immunoglobulin Structure. Immunol Invest 2019;48:771-80. [Crossref] [PubMed]
- Franklin EC, Frangione B. Immunoglobulins. Annu Rev Med 1969;20:155-74. [Crossref] [PubMed]
- Saxon A, Stevens RH, Ashman RF. Regulation of immunoglobulin production in human peripheral bood leukocytes: cellular interactions. J Immunol 1977;118:1782-9. [Crossref] [PubMed]
- Esser C, Radbruch A. Immunoglobulin class switching: molecular and cellular analysis. Annu Rev Immunol 1990;8:717-35. [Crossref] [PubMed]
- Faupel-Badger JM, Hsieh CC, Troisi R, et al. Plasma volume expansion in pregnancy: implications for biomarkers in population studies. Cancer Epidemiol Biomarkers Prev 2007;16:1720-3. [Crossref] [PubMed]
- Schroeder HW Jr, Cavacini L. Structure and function of immunoglobulins. J Allergy Clin Immunol 2010;125:S41-52. [Crossref] [PubMed]
- Hortin GL, Meilinger B. Cross-reactivity of amino acids and other compounds in the biuret reaction: interference with urinary peptide measurements. Clin Chem 2005;51:1411-9. [Crossref] [PubMed]
- Rodríguez-Vico F, Martínez-Cayuela M, García-Peregrín E, et al. A procedure for eliminating interferences in the lowry method of protein determination. Anal Biochem 1989;183:275-8. [Crossref] [PubMed]
- Gupta A, Stockham SL. Negative interference of icteric serum on a bichromatic biuret total protein assay. Vet Clin Pathol 2014;43:422-7. [Crossref] [PubMed]
- Lubran MM. The measurement of total serum proteins by the Biuret method. Ann Clin Lab Sci 1978;8:106-10. [PubMed]
- Peters T Jr, Stewart AJ. Albumin research in the 21st century. Biochim Biophys Acta 2013;1830:5351-3. [Crossref] [PubMed]
- Lyon AW, Meinert P, Bruce GA, et al. Influence of methodology on the detection and diagnosis of congenital analbuminemia. Clin Chem 1998;44:2365-7. [Crossref] [PubMed]
- Boonsriwong W, Chunta S, Thepsimanon N, et al. Thin Film Plastic Antibody-Based Microplate Assay for Human Serum Albumin Determination. Polymers (Basel) 2021;13:1763. [Crossref] [PubMed]
- Cornes MP, Ford C, Gama R. Undetected spurious hypernatraemia wastes health-care resources. Ann Clin Biochem 2011;48:87-8. [Crossref] [PubMed]
- Jolles S, Borrell R, Zouwail S, et al. Calculated globulin (CG) as a screening test for antibody deficiency. Clin Exp Immunol 2014;177:671-8. [Crossref] [PubMed]
- Holding S, Khan S, Sewell WA, et al. Using calculated globulin fraction to reduce diagnostic delay in primary and secondary hypogammaglobulinaemias: results of a demonstration project. Ann Clin Biochem 2015;52:319-26. [Crossref] [PubMed]
- Ogershok PR, Hogan MB, Welch JE, et al. Spectrum of illness in pediatric common variable immunodeficiency. Ann Allergy Asthma Immunol 2006;97:653-6. [Crossref] [PubMed]
- Piza CFST, Aranda CS, Solé D, et al. Serum Protein Electrophoresis May Be Used as a Screening Tool for Antibody Deficiency in Children and Adolescents. Front Immunol 2021;12:712637. [Crossref] [PubMed]
- Buckley RH. Humoral immunodeficiency. Clin Immunol Immunopathol 1986;40:13-24. [Crossref] [PubMed]
- Hall A, Baldwin C, Scott C, et al. Clinical immunology. 2016. Available online: https://global.oup.com/ukhe/product/clinical-immunology-9780199657650?cc=us&lang=en&
- Fritsche HA Jr, De Leon E. The determination of serum immunoglobulins by automated nephelometric analysis. Am J Med Technol 1975;41:136-44. [PubMed]
- Bossuyt X, Blanckaert N. Evaluation of interferences in rate and fixed-time nephelometric assays of specific serum proteins. Clin Chem 1999;45:62-7. [Crossref] [PubMed]
- Zajec M, Langerhorst P, VanDuijn MM, et al. Mass Spectrometry for Identification, Monitoring, and Minimal Residual Disease Detection of M-Proteins. Clin Chem 2020;66:421-33. [Crossref] [PubMed]
- Jenner E. Serum free light chains in clinical laboratory diagnostics. Clin Chim Acta 2014;427:15-20. [Crossref] [PubMed]
- Rajkumar SV, Dimopoulos MA, Palumbo A, et al. International Myeloma Working Group updated criteria for the diagnosis of multiple myeloma. Lancet Oncol 2014;15:e538-48. [Crossref] [PubMed]
- Dispenzieri A, Kyle R, Merlini G, et al. International Myeloma Working Group guidelines for serum-free light chain analysis in multiple myeloma and related disorders. Leukemia 2009;23:215-24. [Crossref] [PubMed]
- Levinson SS. Urine immunofixation electrophoresis remains important and is complementary to serum free light chain. Clin Chem Lab Med 2011;49:1801-4. [Crossref] [PubMed]
- Siegel D, Bilotti E, van Hoeven KH. Serum Free Light Chain Analysis for Diagnosis, Monitoring, and Prognosis of Monoclonal Gammopathies. Laboratory Medicine 2009;40:363-6. [Crossref]
- Jacobs JF, van der Molen RG, Bossuyt X, et al. Antigen excess in modern immunoassays: to anticipate on the unexpected. Autoimmun Rev 2015;14:160-7. [Crossref] [PubMed]
- Jenkins MA. Serum and urine electrophoresis for detection and identification of monoclonal proteins. Clin Biochem Rev 2009;30:119-22. [PubMed]
- Mussap M, Ponchia S, Zaninotto M, et al. Evaluation of a new capillary zone electrophoresis system for the identification and typing of Bence Jones Protein. Clin Biochem 2006;39:152-9. [Crossref] [PubMed]
- Talreja H, Akbari A, White CA, et al. Predicting kidney transplantation outcomes using proteinuria ascertained from spot urine samples versus timed urine collections. Am J Kidney Dis 2014;64:962-8. [Crossref] [PubMed]
- Yamamoto H, Yamamoto K, Yoshida K, et al. Modified Cut-Off Value of the Urine Protein-To-Creatinine Ratio Is Helpful for Identifying Patients at High Risk for Chronic Kidney Disease: Validation of the Revised Japanese Guideline. Tohoku J Exp Med 2015;237:201-7. [Crossref] [PubMed]
- Kamińska J, Dymicka-Piekarska V, Tomaszewska J, et al. Diagnostic utility of protein to creatinine ratio (P/C ratio) in spot urine sample within routine clinical practice. Crit Rev Clin Lab Sci 2020;57:345-64. [Crossref] [PubMed]
- National Institute of Health and Care Excellence. Chronic kidney disease: assessment and management NICE guideline [NG203]. 2021. Available online: https://www.nice.org.uk/guidance/ng203
- Busby DE, Bakris GL. Comparison of commonly used assays for the detection of microalbuminuria. J Clin Hypertens (Greenwich) 2004;6:8-12. [Crossref] [PubMed]
- Stevens PE, Levin A. Kidney Disease: Improving Global Outcomes Chronic Kidney Disease Guideline Development Work Group Members. Evaluation and management of chronic kidney disease: synopsis of the kidney disease: improving global outcomes 2012 clinical practice guideline. Ann Intern Med 2013;158:825-30. [Crossref] [PubMed]
- Prasad RM, Tikaria R. Microalbuminuria. Treasure Island (FL): StatPearls Publishing, 2022.
- Andrassy KM. Comments on 'KDIGO 2012 Clinical Practice Guideline for the Evaluation and Management of Chronic Kidney Disease'. Kidney Int 2013;84:622-3. [Crossref] [PubMed]
- Chau TN, Mok MY, Chan EY, et al. Evaluation of performance of measurement of faecal α(1)-antitrypsin clearance and technetium-99m human serum albumin scintigraphy in protein-losing enteropathy. Digestion 2011;84:199-206. [Crossref] [PubMed]
- Braamskamp MJ, Dolman KM, Tabbers MM. Clinical practice. Protein-losing enteropathy in children. Eur J Pediatr 2010;169:1179-85. [Crossref] [PubMed]
- Strygler B, Nicar MJ, Santangelo WC, et al. Alpha 1-antitrypsin excretion in stool in normal subjects and in patients with gastrointestinal disorders. Gastroenterology 1990;99:1380-7. [Crossref] [PubMed]
- Skarżyńska E, Kiersztyn B, Wilczyńska P, et al. Total proteolytic activity and concentration of alpha-1 antitrypsin in meconium for assessment of the protease/antiprotease balance. Eur J Obstet Gynecol Reprod Biol 2018;223:133-8. [Crossref] [PubMed]
- Choudhary S, Gibson PR, Deacon MC, et al. Measurement of faecal alpha 1-antitrypsin: methodologies and clinical application. J Gastroenterol Hepatol 1996;11:311-8. [Crossref] [PubMed]
- Rao PS. Protein-losing enteropathy following the Fontan operation. J Invasive Cardiol 2007;19:447-8. [PubMed]
- Zheng WJ, Tian XP, Li L, et al. Protein-losing enteropathy in systemic lupus erythematosus: analysis of the clinical features of fifteen patients. J Clin Rheumatol 2007;13:313-6. [Crossref] [PubMed]
- Elli L, Topa M, Rimondi A. Protein-losing enteropathy. Curr Opin Gastroenterol 2020;36:238-44. [Crossref] [PubMed]
- Stretti L, Zippo D, Coats AJS, et al. A year in heart failure: an update of recent findings. ESC Heart Fail 2021;8:4370-93. [Crossref] [PubMed]
- Karabulut A, Kaplan A, Aslan C, et al. The association between NT-proBNP levels, functional capacity and stage in patients with heart failure. Acta Cardiol 2005;60:631-8. [Crossref] [PubMed]
- Fazal IA, Bhagra SK, Bailey KM, et al. Impact of using different guideline recommended serum natriuretic peptide thresholds on the diagnosis and referral rates of a diagnostic heart failure clinic. Int J Clin Pract 2015;69:1349-56. [Crossref] [PubMed]
- Authors/Task Force Members. 2021 ESC Guidelines for the diagnosis and treatment of acute and chronic heart failure: Developed by the Task Force for the diagnosis and treatment of acute and chronic heart failure of the European Society of Cardiology (ESC). With the special contribution of the Heart Failure Association (HFA) of the ESC. Eur J Heart Fail 2022;24:4-131. [Crossref] [PubMed]
- Tate J, Ward G. Interferences in immunoassay. Clin Biochem Rev 2004;25:105-20. [PubMed]
- Baggish AL, van Kimmenade RR, Januzzi JL Jr. The differential diagnosis of an elevated amino-terminal pro-B-type natriuretic peptide level. Am J Cardiol 2008;101:43-8. [Crossref] [PubMed]
- Maries L, Manitiu I. Diagnostic and prognostic values of B-type natriuretic peptides (BNP) and N-terminal fragment brain natriuretic peptides (NT-pro-BNP). Cardiovasc J Afr 2013;24:286-9. [Crossref] [PubMed]
- Huang LL, Xia HH, Zhu SL. Ascitic Fluid Analysis in the Differential Diagnosis of Ascites: Focus on Cirrhotic Ascites. J Clin Transl Hepatol 2014;2:58-64. [PubMed]
- Gokturk HS, Demir M, Ozturk NA, et al. The role of ascitic fluid viscosity in the differential diagnosis of ascites. Can J Gastroenterol 2010;24:255-9. [Crossref] [PubMed]
- Chubb SP, Williams RA. Biochemical Analysis of Pleural Fluid and Ascites. Clin Biochem Rev 2018;39:39-50. [PubMed]
- Berg J. The UK Pathology Harmony initiative; The foundation of a global model. Clin Chim Acta 2014;432:22-6. [Crossref] [PubMed]
- Arif SK, Verheij J, Groeneveld AB, et al. Hypoproteinemia as a marker of acute respiratory distress syndrome in critically ill patients with pulmonary edema. Intensive Care Med 2002;28:310-7. [Crossref] [PubMed]
- Gómez-Cantarino S, Agulló-Ortuño MT, de Dios-Aguado M, et al. Prevalence of Hypoproteinemia and Hypoalbuminemia in Pregnant Women from Three Different Socioeconomic Populations. Int J Environ Res Public Health 2020;17:6275. [Crossref] [PubMed]
- Yuwen P, Chen W, Lv H, et al. Albumin and surgical site infection risk in orthopaedics: a meta-analysis. BMC Surg 2017;17:7. [Crossref] [PubMed]
- Vincent JL, Dubois MJ, Navickis RJ, et al. Hypoalbuminemia in acute illness: is there a rationale for intervention? A meta-analysis of cohort studies and controlled trials. Ann Surg 2003;237:319-34. [Crossref] [PubMed]
- Nipper CA, Lim K, Riveros C, et al. The Association between Serum Albumin and Post-Operative Outcomes among Patients Undergoing Common Surgical Procedures: An Analysis of a Multi-Specialty Surgical Cohort from the National Surgical Quality Improvement Program (NSQIP). J Clin Med 2022;11:6543. [Crossref] [PubMed]
- Finfer S, Bellomo R, Boyce N, et al. A comparison of albumin and saline for fluid resuscitation in the intensive care unit. N Engl J Med 2004;350:2247-56. [Crossref] [PubMed]
- Caironi P, Tognoni G, Masson S, et al. Albumin replacement in patients with severe sepsis or septic shock. N Engl J Med 2014;370:1412-21. [Crossref] [PubMed]
- Gabay C, Kushner I. Acute-phase proteins and other systemic responses to inflammation. N Engl J Med 1999;340:448-54. [Crossref] [PubMed]
- Grover Z, Ee LC. Protein energy malnutrition. Pediatr Clin North Am 2009;56:1055-68. [Crossref] [PubMed]
- Pham TP, Alou MT, Golden MH, et al. Difference between kwashiorkor and marasmus: Comparative meta-analysis of pathogenic characteristics and implications for treatment. Microb Pathog 2021;150:104702. [Crossref] [PubMed]
- Scrimshaw NS, Viteri FE. INCAP studies of kwashiorkor and marasmus. Food Nutr Bull 2010;31:34-41. [Crossref] [PubMed]
- Chen XD, Wang YF, Wang YL, et al. Induced Hyperproteinemia and Its Effects on the Remodeling of Fat Bodies in Silkworm, Bombyx mori. Front Physiol 2018;9:302. [Crossref] [PubMed]
- Keller U. Nutritional Laboratory Markers in Malnutrition. J Clin Med 2019;8:775. [Crossref] [PubMed]
- Smith G, Robinson PH, Fleck A. Serum albumin distribution in early treated anorexia nervosa. Nutrition 1996;12:677-84. [Crossref] [PubMed]
- Brock F, Bettinelli LA, Dobner T, et al. Prevalence of hypoalbuminemia and nutritional issues in hospitalized elders. Rev Lat Am Enfermagem 2016;24:e2736. [Crossref] [PubMed]
- Soeters PB, Wolfe RR, Shenkin A. Hypoalbuminemia: Pathogenesis and Clinical Significance. JPEN J Parenter Enteral Nutr 2019;43:181-93. [Crossref] [PubMed]
- Litao MK, Kamat D. Erythrocyte sedimentation rate and C-reactive protein: how best to use them in clinical practice. Pediatr Ann 2014;43:417-20. [Crossref] [PubMed]
- Weinstein PD, Doerfler ME. Systemic complications of fluid resuscitation. Crit Care Clin 1992;8:439-48. [Crossref] [PubMed]
- Carvalho JR, Verdelho Machado M. New Insights About Albumin and Liver Disease. Ann Hepatol 2018;17:547-60. [Crossref] [PubMed]
- Newsome PN, Cramb R, Davison SM, et al. Guidelines on the management of abnormal liver blood tests. Gut 2018;67:6-19. [Crossref] [PubMed]
- Kütting F, Schubert J, Franklin J, et al. Insufficient evidence of benefit regarding mortality due to albumin substitution in HCC-free cirrhotic patients undergoing large volume paracentesis. J Gastroenterol Hepatol 2017;32:327-38. [Crossref] [PubMed]
- Chen Q, Lu M, Monks BR, et al. Insulin Is Required to Maintain Albumin Expression by Inhibiting Forkhead Box O1 Protein. J Biol Chem 2016;291:2371-8. [Crossref] [PubMed]
- Caridi G, Lugani F, Angeletti A, et al. Variations in the Human Serum Albumin Gene: Molecular and Functional Aspects. Int J Mol Sci 2022;23:1159. [Crossref] [PubMed]
- Toye JM, Lemire EG, Baerg KL. Perinatal and childhood morbidity and mortality in congenital analbuminemia. Paediatr Child Health 2012;17:e20-3. [PubMed]
- Minchiotti L, Caridi G, Campagnoli M, et al. Diagnosis, Phenotype, and Molecular Genetics of Congenital Analbuminemia. Front Genet 2019;10:336. [Crossref] [PubMed]
- Staps P, Hogeveen M, Fuijkschot J, et al. Understanding fetal factors that contribute to preterm birth: Sjögren-Larsson syndrome as a model. J Perinat Med 2018;46:523-9. [Crossref] [PubMed]
- Ungaro R, Babyatsky MW, Zhu H, et al. Protein-losing enteropathy in ulcerative colitis. Case Rep Gastroenterol 2012;6:177-82. [Crossref] [PubMed]
- Collins MH, Capocelli K, Yang GY. Eosinophilic Gastrointestinal Disorders Pathology. Front Med (Lausanne) 2018;4:261. [Crossref] [PubMed]
- Awazawa R, Yamamoto Y, Mine Y, et al. Systemic lupus erythematosus complicated with protein-losing enteropathy: a case report and review of the published works. J Dermatol 2012;39:454-61. [Crossref] [PubMed]
- Umar SB, DiBaise JK. Protein-losing enteropathy: case illustrations and clinical review. Am J Gastroenterol 2010;105:43-9; quiz 50. [Crossref] [PubMed]
- Craven MD, Washabau RJ. Comparative pathophysiology and management of protein-losing enteropathy. J Vet Intern Med 2019;33:383-402. [Crossref] [PubMed]
- D'Amico G, Bazzi C. Pathophysiology of proteinuria. Kidney Int 2003;63:809-25. [Crossref] [PubMed]
- Cil O, Perwad F. Monogenic Causes of Proteinuria in Children. Front Med (Lausanne) 2018;5:55. [Crossref] [PubMed]
- Kooman JP, van der Sande FM. Body Fluids in End-Stage Renal Disease: Statics and Dynamics. Blood Purif 2019;47:223-9. [Crossref] [PubMed]
- Kaysen GA, Gambertoglio J, Jimenez I, et al. Effect of dietary protein intake on albumin homeostasis in nephrotic patients. Kidney Int 1986;29:572-7. [Crossref] [PubMed]
- Don BR, Kaysen G. Serum albumin: relationship to inflammation and nutrition. Semin Dial 2004;17:432-7. [Crossref] [PubMed]
- Baynes JW, Thorpe SR. Identification of the sites of albumin catabolism in the rat. Arch Biochem Biophys 1981;206:372-9. [Crossref] [PubMed]
- Committee JF. British National Formulary. London: British Medical Association and Royal Pharmaceutical Society of Great Britain 2022 [updated 19/12/2022]. Available online: https://bnf.nice.org.uk/
- Flament J, Jelti L, Lok C, et al. Severe dapsone-induced hypoalbuminemia in a case of mucous membrane pemphigoid. JAAD Case Rep 2020;6:1098-100. [Crossref] [PubMed]
- Strickler JH, LoRusso P, Salgia R, et al. Phase I Dose-Escalation and -Expansion Study of Telisotuzumab (ABT-700), an Anti-c-Met Antibody, in Patients with Advanced Solid Tumors. Mol Cancer Ther 2020;19:1210-7. [Crossref] [PubMed]
- Cirillo E, Cancrini C, Azzari C, et al. Clinical, Immunological, and Molecular Features of Typical and Atypical Severe Combined Immunodeficiency: Report of the Italian Primary Immunodeficiency Network. Front Immunol 2019;10:1908. [Crossref] [PubMed]
- Mims RB, Lopez RJ, Finck UA, et al. Hypoglobulinemia in acromegaly. Horm Metab Res 1976;8:295-8. [Crossref] [PubMed]
- Gitlin D, Gross PA, Janeway CA. The gamma globulins and their clinical significance. II. Hypogammaglobulinemia. N Engl J Med 1959;260:72-6. [Crossref] [PubMed]
- Wright PE, Sears DA. Hypogammaglobulinemia and pernicious anemia. South Med J 1987;80:243-6. [Crossref] [PubMed]
- Yazdani R, Habibi S, Sharifi L, et al. Common Variable Immunodeficiency: Epidemiology, Pathogenesis, Clinical Manifestations, Diagnosis, Classification, and Management. J Investig Allergol Clin Immunol 2020;30:14-34. [Crossref] [PubMed]
- Globig AM, Strohmeier V, Surabattula R, et al. Evaluation of Laboratory and Sonographic Parameters for Detection of Portal Hypertension in Patients with Common Variable Immunodeficiency. J Clin Immunol 2022;42:1626-37. [Crossref] [PubMed]
- Kalha I, Sellin JH. Common variable immunodeficiency and the gastrointestinal tract. Curr Gastroenterol Rep 2004;6:377-83. [Crossref] [PubMed]
- Sève P, Bourdillon L, Sarrot-Reynauld F, et al. Autoimmune hemolytic anemia and common variable immunodeficiency: a case-control study of 18 patients. Medicine (Baltimore) 2008;87:177-84. [Crossref] [PubMed]
- Tak Manesh A, Azizi G, Heydari A, et al. Epidemiology and pathophysiology of malignancy in common variable immunodeficiency? Allergol Immunopathol (Madr) 2017;45:602-15. [Crossref] [PubMed]
- Huq M, Bhatnagar NK, Hostoffer RW. Hypogammaglobulinemia. 2023.
- Stiehm ER. Humoral immunity in malnutrition. Fed Proc 1980;39:3093-7. [PubMed]
- Griffin TA, Hostoffer RW, Tserng KY, et al. Parathyroid hormone resistance and B cell lymphopenia in propionic acidemia. Acta Paediatr 1996;85:875-8. [Crossref] [PubMed]
- Land MH, Roberts RL. Neutropenia and Hypogammaglobulinemia in Propionic Acidemia. J Allergy Clin Immunol 2006;117:S172. [Crossref]
- Limdi JK, Hyde GM. Evaluation of abnormal liver function tests. Postgrad Med J 2003;79:307-12. [Crossref] [PubMed]
- Kumar V, Jarzabek-Chorzelska M, Sulej J, et al. Celiac disease and immunoglobulin a deficiency: how effective are the serological methods of diagnosis? Clin Diagn Lab Immunol 2002;9:1295-300. [PubMed]
- Elson CO, James SP, Graeff AS, et al. Hypogammaglobulinemia due to abnormal suppressor T-cell activity in Crohn's disease. Gastroenterology 1984;86:569-76. [Crossref] [PubMed]
- Heaney JLJ, Campbell JP, Iqbal G, et al. Characterisation of immunoparesis in newly diagnosed myeloma and its impact on progression-free and overall survival in both old and recent myeloma trials. Leukemia 2018;32:1727-38. [Crossref] [PubMed]
- Federspil G, Vettor R, Bossi A, et al. Hypoproteinemia in acromegaly. J Endocrinol Invest 1979;2:217-9. [Crossref] [PubMed]
- Knight AK, Cunningham-Rundles C. Oxcarbazepine-induced immunoglobulin deficiency. Clin Diagn Lab Immunol 2005;12:560-1. [PubMed]
- Otani IM, Lehman HK, Jongco AM, et al. Practical guidance for the diagnosis and management of secondary hypogammaglobulinemia: A Work Group Report of the AAAAI Primary Immunodeficiency and Altered Immune Response Committees. J Allergy Clin Immunol 2022;149:1525-60. [Crossref] [PubMed]
- Paricaud K, Moulis G, Combis MS, et al. Causes of protidemia above 100g/L. Eur J Intern Med 2014;25:e123. [Crossref] [PubMed]
- Steinberger BA, Ford SM, Coleman TA. Intravenous immunoglobulin therapy results in post-infusional hyperproteinemia, increased serum viscosity, and pseudohyponatremia. Am J Hematol 2003;73:97-100. [Crossref] [PubMed]
- Leung N, Bridoux F, Batuman V, et al. The evaluation of monoclonal gammopathy of renal significance: a consensus report of the International Kidney and Monoclonal Gammopathy Research Group. Nat Rev Nephrol 2019;15:45-59. [Crossref] [PubMed]
- Marinkovic A, Zypchen LN, Chan J, et al. Monoclonal gammopathy of clinical significance: what the rheumatologist needs to know. Lancet Rheumatol 2022;4:e362-e373. [Crossref]
- Dispenzieri A. Monoclonal gammopathies of clinical significance. Hematology Am Soc Hematol Educ Program 2020;2020:380-8. [Crossref] [PubMed]
- Perez Rogers A, Estes M. Hyperviscosity Syndrome. Treasure Island (FL): StatPearls Publishing; 2023.
- Florova B, Rajdl D, Racek J, et al. NGAL, albumin and cystatin C during cisplatin therapy. Physiol Res 2020;69:307-17. [Crossref] [PubMed]
- Mesquita RB, Rangel AO. A sequential injection system for the spectrophotometric determination of calcium, magnesium and alkalinity in water samples. Anal Sci 2004;20:1205-10. [Crossref] [PubMed]
- Masaki T, Matsuura T, Ohkawa K, et al. All-trans retinoic acid down-regulates human albumin gene expression through the induction of C/EBPbeta-LIP. Biochem J 2006;397:345-53. [Crossref] [PubMed]
- Mutlu EA, Keshavarzian A, Mutlu GM. Hyperalbuminemia and elevated transaminases associated with high-protein diet. Scand J Gastroenterol 2006;41:759-60. [Crossref] [PubMed]
- Hoo T, Lim EM, John M, et al. Calculated globulin as a screening tool for hypogammaglobulinaemia or paraproteins in hospitalized patients. Ann Clin Biochem 2021;58:236-43. [Crossref] [PubMed]
- Hughes M, Davidson DF, McColl M. Outcomes of discretionary laboratory requesting of serum protein electrophoresis. Ann Clin Biochem 2006;43:372-4. [Crossref] [PubMed]
- Whicher JT, Calvin J, Riches P, et al. The laboratory investigation of paraproteinaemia. Ann Clin Biochem 1987;24:119-32. [Crossref] [PubMed]
- Uchida K, Kusuda T, Koyabu M, et al. Regulatory T cells in type 1 autoimmune pancreatitis. Int J Rheumatol 2012;2012:795026. [Crossref] [PubMed]
- Flynn S, Eisenstein S. Inflammatory Bowel Disease Presentation and Diagnosis. Surg Clin North Am 2019;99:1051-62. [Crossref] [PubMed]
- Zhao EJ, Cheng CV, Mattman A, et al. Polyclonal hypergammaglobulinaemia: assessment, clinical interpretation, and management. Lancet Haematol 2021;8:e365-75. [Crossref] [PubMed]
- Kucharzik T, Koletzko S, Kannengiesser K, et al. Ulcerative Colitis-Diagnostic and Therapeutic Algorithms. Dtsch Arztebl Int 2020;117:564-74. [PubMed]
- Ayling RM, Kok K. Fecal Calprotectin. Adv Clin Chem 2018;87:161-90. [Crossref] [PubMed]
- Connelly K, Morand EF. Systemic lupus erythematosus: a clinical update. Intern Med J 2021;51:1219-28. [Crossref] [PubMed]
- Dispenzieri A, Gertz MA, Therneau TM, et al. Retrospective cohort study of 148 patients with polyclonal gammopathy. Mayo Clin Proc 2001;76:476-87. [Crossref] [PubMed]
- Lanzillotta M, Mancuso G, Della-Torre E. Advances in the diagnosis and management of IgG4 related disease. BMJ 2020;369:m1067. [Crossref] [PubMed]
- Harkness T, Fu X, Zhang Y, et al. Immunoglobulin G and immunoglobulin G subclass concentrations differ according to sex and race. Ann Allergy Asthma Immunol 2020;125:190-195.e2. [Crossref] [PubMed]
- Dimeski G, Hamer A, Cooper C, et al. Pseudohypophosphataemia secondary to paraproteinaemia may occur without the presence of hypergammaglobulinaemia. Pathology 2016;48:102-3. [Crossref] [PubMed]
- Mouallem M, Antipov N, Mayan H, et al. Hyperglobulinemia in amiodarone-induced pneumonitis. Cardiovasc Drugs Ther 2007;21:63-7. [Crossref] [PubMed]
- Alla V, Abraham J, Siddiqui J, et al. Autoimmune hepatitis triggered by statins. J Clin Gastroenterol 2006;40:757-61. [Crossref] [PubMed]
- Sheldon J, Wheeler RD, Powles R. Electrophoretic patterns post daratumumab. Ann Clin Biochem 2018;55:299-301. [Crossref] [PubMed]
- Antonucci R, Zaffanello M, Puxeddu E, et al. Use of non-steroidal anti-inflammatory drugs in pregnancy: impact on the fetus and newborn. Curr Drug Metab 2012;13:474-90. [Crossref] [PubMed]
- Damase-Michel C, Hurault-Delarue C. Ibuprofen does not seem to increase global malformation risk but NSAID use in late pregnancy remains a concern. Evid Based Med 2014;19:74. [Crossref] [PubMed]
- Cravedi P, Remuzzi G. Pathophysiology of proteinuria and its value as an outcome measure in chronic kidney disease. Br J Clin Pharmacol 2013;76:516-23. [PubMed]
- Lönnerdal B, Forsum E, Hambraeus L. A longitudinal study of the protein, nitrogen, and lactose contents of human milk from Swedish well-nourished mothers. Am J Clin Nutr 1976;29:1127-33. [Crossref] [PubMed]
- Torer B, Hanta D, Yapakci E, et al. Association of Serum Albumin Level and Mortality in Premature Infants. J Clin Lab Anal 2016;30:867-72. [Crossref] [PubMed]
- Burki M, Yousaf M, Orakzai SA. Serum proteins electrophoresis in infants and children. J Pak Med Assoc 1996;46:252-5. [PubMed]
- Adeli K, Higgins V, Trajcevski K, et al. The Canadian laboratory initiative on pediatric reference intervals: A CALIPER white paper. Crit Rev Clin Lab Sci 2017;54:358-413. [Crossref] [PubMed]
- Ziegler KB, Muzzio DO, Matzner F, et al. Human pregnancy is accompanied by modifications in B cell development and immunoglobulin profile. J Reprod Immunol 2018;129:40-7. [Crossref] [PubMed]
- Aagaard-Tillery KM, Silver R, Dalton J. Immunology of normal pregnancy. Semin Fetal Neonatal Med 2006;11:279-95. [Crossref] [PubMed]
- Benster B, Wood EJ. Immunoglobulin levels in normal pregnancy and pregnancy complicated by hypertension. J Obstet Gynaecol Br Commonw 1970;77:518-22. [Crossref] [PubMed]
- Garcia-Prat M, Vila-Pijoan G, Martos Gutierrez S, et al. Age-specific pediatric reference ranges for immunoglobulins and complement proteins on the Optilite(™) automated turbidimetric analyzer. J Clin Lab Anal 2018;32:e22420. [Crossref] [PubMed]
- Kutukculer N, Karaca NE, Demircioglu O, et al. Increases in serum immunoglobulins to age-related normal levels in children with IgA and/or IgG subclass deficiency. Pediatr Allergy Immunol 2007;18:167-73. [Crossref] [PubMed]
- Kanariou M, Petridou E, Liatsis M, et al. Age patterns of immunoglobulins G, A & M in healthy children and the influence of breast feeding and vaccination status. Pediatr Allergy Immunol 1995;6:24-9. [Crossref] [PubMed]
- Steinfeld JL, Davidson JD, Gordon RS Jr, et al. The mechanism of hypoproteinemia in patients with regional enteritis and ulcerative colitis. Am J Med 1960;29:405-15. [Crossref] [PubMed]
- Solomons NW, Wagonfeld JB, Rieger C, et al. Some biochemical indices of nutrition in treated cystic fibrosis patients. Am J Clin Nutr 1981;34:462-74. [Crossref] [PubMed]
- Ozaslan E, Köseoğlu T, Kayhan B. Coeliac crisis in adults: report of two cases. Eur J Emerg Med 2004;11:363-5. [Crossref] [PubMed]
- Tian CR, Qian L, Shen XZ, et al. Distribution of serum total protein in elderly Chinese. PLoS One 2014;9:e101242. [Crossref] [PubMed]
- Schwarz S, Prokopchuk O, Esefeld K, et al. The clinical picture of cachexia: a mosaic of different parameters (experience of 503 patients). BMC Cancer 2017;17:130. [Crossref] [PubMed]
- Stinco M, Montemaggi A, Noccioli B, et al. An Unusual Case of Hypoproteinemia in Childhood: Keep in Mind Trichobezoar. Front Pediatr 2020;8:82. [Crossref] [PubMed]
- Wen J, Chen X, Wei S, et al. Research Progress and Treatment Status of Liver Cirrhosis with Hypoproteinemia. Evid Based Complement Alternat Med 2022;2022:2245491. [Crossref] [PubMed]
Cite this article as: Duvall LE, Shipman AR, Shipman KE. Investigative algorithms for disorders affecting plasma proteins with a focus on albumin and the calculated globulin fraction: a narrative review. J Lab Precis Med 2023;8:19.